The FLOWER project aims at producing 3 prototypes of biocomposites in the nautical sector (a foilboat), the publicity sector (an advertising support) and the automotive one (a headliner). It is now done, with the three prototypes finalised; a description of our previous work can be found here. Following the acceptance of a project extension, 3 new prototypes were produced (a wind turbine, a nautical dinghy and a refrigerated truck panel).
The innovation in these prototypes comes from the use of natural fibres to replace glass fibre. The flax fibre used in the FLOWER project is lighter, has a low environmental impact and is locally produced. However, this natural fibre is subject to climatic conditions and certain diseases. In order to optimise the properties of the fibres used in biocomposite materials, work with flax fibre producers is needed to identify the best fibres and harvesting periods. This involves analysing batches of fibres to better understand their performance.
In this study, the partner Teillage Vandecandelaere provided batches of fibre grown on different soils, with different varieties and with different final fibre qualities, potentially induced by cultivation practices, diseases or periods of drought experienced by the plants. In particular, the consequences of drought on fibre performance were analysed; this issue is particularly important in the context of global warming.
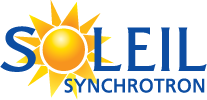
In order to be able to analyse the ultrastructure and intrinsic properties of flax fibres in detail, the use of large instruments and in particular Synchrotrons is a major asset. Two Synchrotrons are located in France, one of which, the SOLEIL Synchrotron radiation centre, is located on the Saclay plateau in Saint Aubin (91). More concretely, it is a particle accelerator (electrons) that produces synchrotron radiation, an extremely bright light that can be used to explore inert or living matter. SOLEIL is a light source with exceptional properties that are necessary for the scientific and industrial communities. It opens up new perspectives for probing matter with a spatial resolution of up to ten nanometres and sensitivity to all types of materials.
The FLOWER partners have therefore made several visits to the Synchrotron SOLEIL to carry out measurements, on the one hand to test the performance and biochemical composition of flax fibres following climatic hazards and on the other hand to check their damage and durability following mechanical or environmental stresses. In addition, Alessia Melelli’s thesis work at Soleil enabled the development of original characterisation techniques applied to flax fibres.
Impact of water stress on flax fibres, DISCO beamline[1]
Initially, work was carried out within the framework of Alessia Melelli’s thesis and is part of the Technical Work Packages 1 and 3. Her thesis was directed by IRDL, a research laboratory of the University of South Brittany, by Alain Bourmaud (IRDL/UBS), and co-supervised by Johnny Beaugrand (INRAe) and Frédéric Jamme (Synchrotron SOLEIL). The general objective of the work was to finely characterise the plant walls of flax fibres in order to better understand the influence of their microstructure and composition on mechanical performance. It was directly linked to one of the major issues of FLOWER, which aims to select optimal flax fibres for the production of high-performance biocomposites.
The experiments conducted at the Synchrotron SOLEIL aimed to gain a better understanding of the structural parameters of flax fibres following a lack of water. They focused on the physical microstructure of these fibres but also on their biochemical composition. The aim of this part of Alessia Melelli’s thesis work was therefore to establish the link between choices made at the farmer level or imposed by the climate and the performance of future biocomposites made with flax fibres. Figure 1 shows an illustration of two batches of flax of the same variety, one grown under normal conditions and the other under water stress.
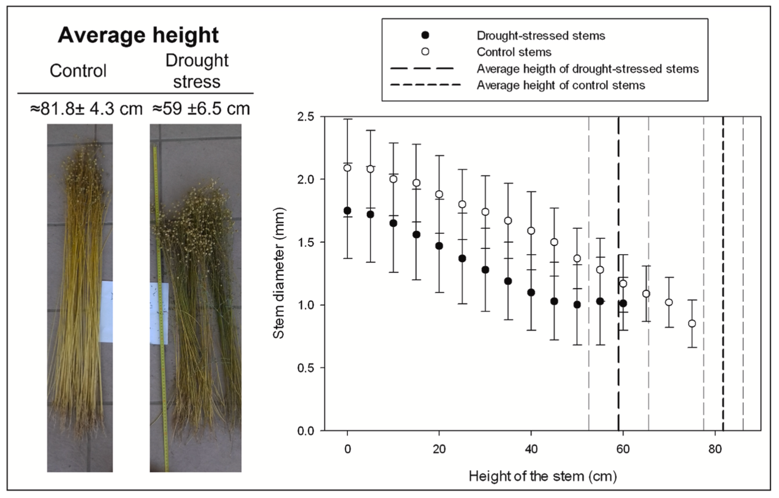
Figure 1. Illustration and measurement of diameter and height of flax stems grown under normal and water stress conditions
The biochemical composition of stressed and unstressed fibres was studied using the UV microscopy equipment of the DISCO beam line at the Synchrotron Soleil. This high-resolution microscopy makes it possible to determine the biochemical composition of flax walls and, above all, to obtain maps of the constituents according to the cultivation or environmental conditions. The interest of this microscopy at SOLEIL is the access, thanks to the unique light flux, to much wider wavelengths than those accessible in conventional laboratories. This allows a wider range of constituents to be identified. Significant differences, which may have an impact on the performance of flax fibres, were found (Figure 2).
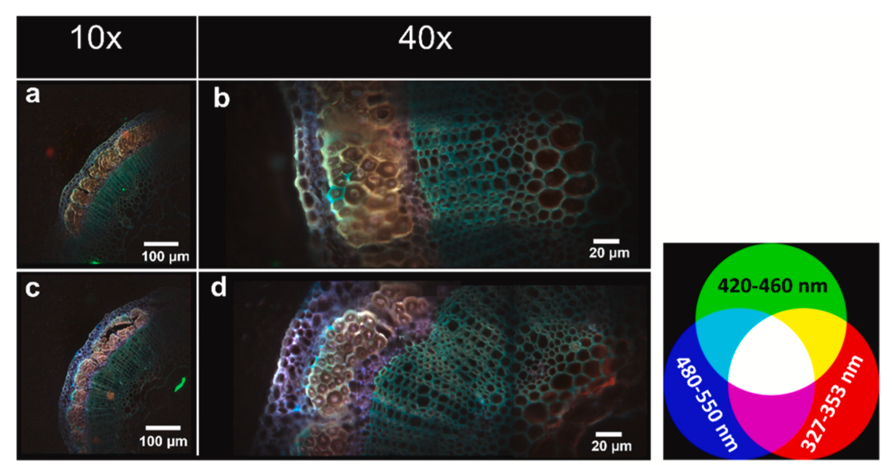
Figure 2. Colour visualization of the differences in biochemical composition in a stressed and unstressed flax stem, each colour representing a family of constituents
Access to this equipment was an opportunity for our consortium and allowed us to increase our knowledge of flax fibre and above all to select high-performance fibres for future biocomposites produced within and outside FLOWER. From now on, these different results can benefit fibre producers who can thus adapt their cultivation conditions to optimise the performance of the fibres, but also the composites industry, whether within or outside the consortium. The latter can select fibres with the best performance for the production of composites. The work resulting from this research has been published and is freely available on the project website.
Development of a new method for measuring the microfibrillar angle of flax fibres, DISCO beamline
Experiments were carried out using a light sheet microscope and the second harmonic generation (SHG) technique; this technology, unique in Europe, allows access to the orientations of matter in very small objects. It can be used to determine the micro-fibrillar angles of the cellulose present in the flax walls, which greatly condition the mechanical performance of the fibres. The fibres from the different batches were analysed and their micro-fibrillar angles determined in order to select the best performing ones. This work made it possible to develop an original, visual and simple method for determining this major parameter of the structure of flax fibres. Figure 3 illustrates these measurements.
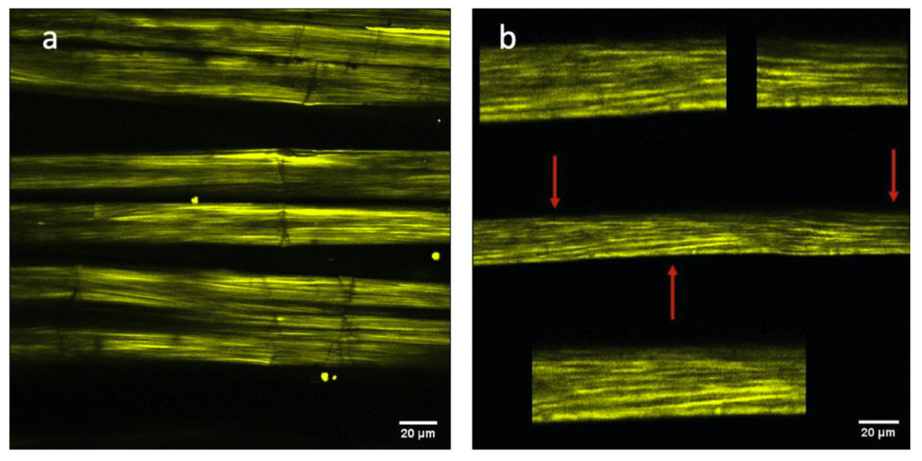
Figure 3. Visualisation of cellulose orientations within flax fibres by SHG microscopy
The knowledge and determination of these angles is of major interest for the composites industry, as their value directly impacts the mechanical properties of the fibres and therefore those of the composite materials. These developments were also carried out in the thesis of Alessia Melelli.
Damage to flax fibres and the influence of their defects, SWING, DISCO and ANATOMIX beamlines
In line with this work, a thesis on the damage of flax fibres/beams and the implications at the composite scale has been financed. INRAE in Nantes recruited Emmanuelle Richely to work on these subjects, under the joint supervision of the University of South Brittany and Teillage Vandecandelaère. The trials made it possible to obtain initial data on the samples from Teillage Vandecandelaère and to plan a future characterisation campaign, in conjunction with Work Package T1.
Several scientific stays to the Synchrotron SOLEIL were necessary in order to carry out the work of the thesis and the post-doctorate that followed. The objective of these trips was to complete experiments in structural characterisation of flax fibres and bundles in order to feed a numerical model. This was made possible by X-ray diffraction tests on the SWING line of the Synchrotron Soleil. Experimental test protocols were developed as part of the project (Figure 4), environmental control systems and specific tensile tools were implemented through a partnership between UoP, INRAE, UBS and SOLEIL. This was also an excellent opportunity to further raise the profile of our project in the national academic scientific community given the degree of innovation of this work.
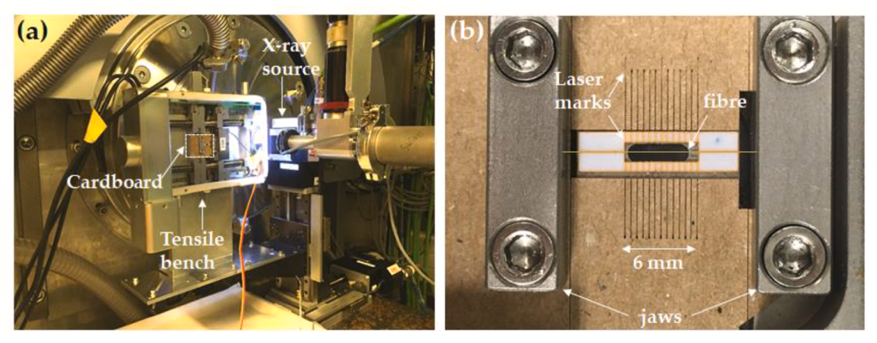
Figure 4. Developments dedicated to diffraction tests on flax fibres on the SWING line
The tests provided information on failure modes and deformations as well as a better understanding of the role of cellulose microfibril orientation, defects and lumens in the failure of linen fibres and bundles. These came from batches from the Vandecandelaère scutching plant that were contrasted in terms of quality and quantity of defects. These data were used to enrich the numerical model representing the mechanical behaviour of flax fibres and bundles developed between INRAE and TVDC. A major originality was the implementation of tensile tests coupled with X-ray diffraction tests, on single fibres and under controlled environmental conditions. We were able to demonstrate the importance of minimising fibre defects to improve fibre performance.
Following this work, measurements of microfibrillar angles were also carried out in the framework of Alessia Melelli’s thesis on defect zones in order to confirm the results obtained on SWING. New SHG measurements carried out with the DISCO line have made it possible to accurately determine the angle values in the fibre defects (Figure 5).
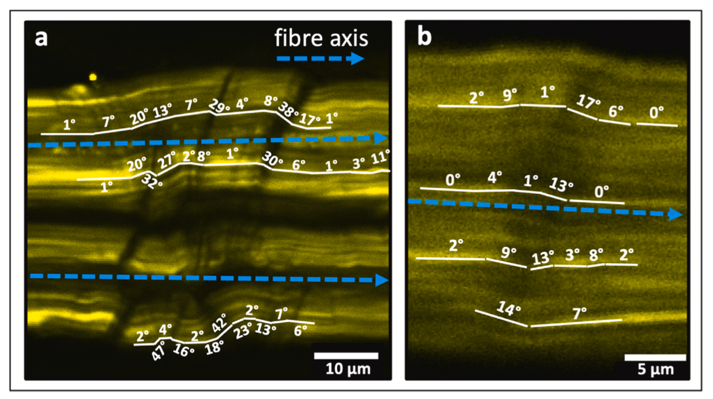
Figure 5. Orientation of cellulose measured by SHG in flax fibres
This work was carried out on modern flax but also on ancient Egyptian flax, thanks to a collaboration with the Louvre Museum,. The study of these ancient objects allows us to better understand the long-term ageing of fibres. During this part of the project, we had the opportunity to work on mortuary fabrics from the Middle Egyptian period, dating back about 4,000 years. An illustration is provided in Figure 6.
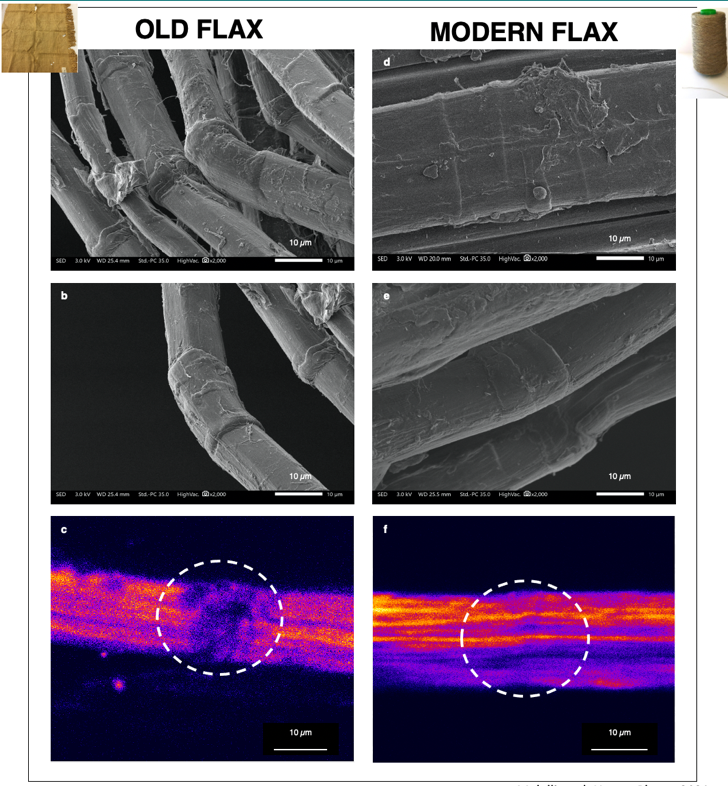
Figure 6. Flax fibre defects observed by electron microscopy and SHG at Soleil
This work is still continuing in the framework of FLOWER, through the post-doc of Camille Goudenhooft at UBS. Since the end of Alessia Melelli’s thesis, we have continued to explore these defect zones, in particular through investigations on the DISCO line but also in nano-tomography, on the ANATOMIX beamline. These tests allow us to visualise the fibres and their defects in 3D, without altering the fibres. An illustration is shown in Figure 7.
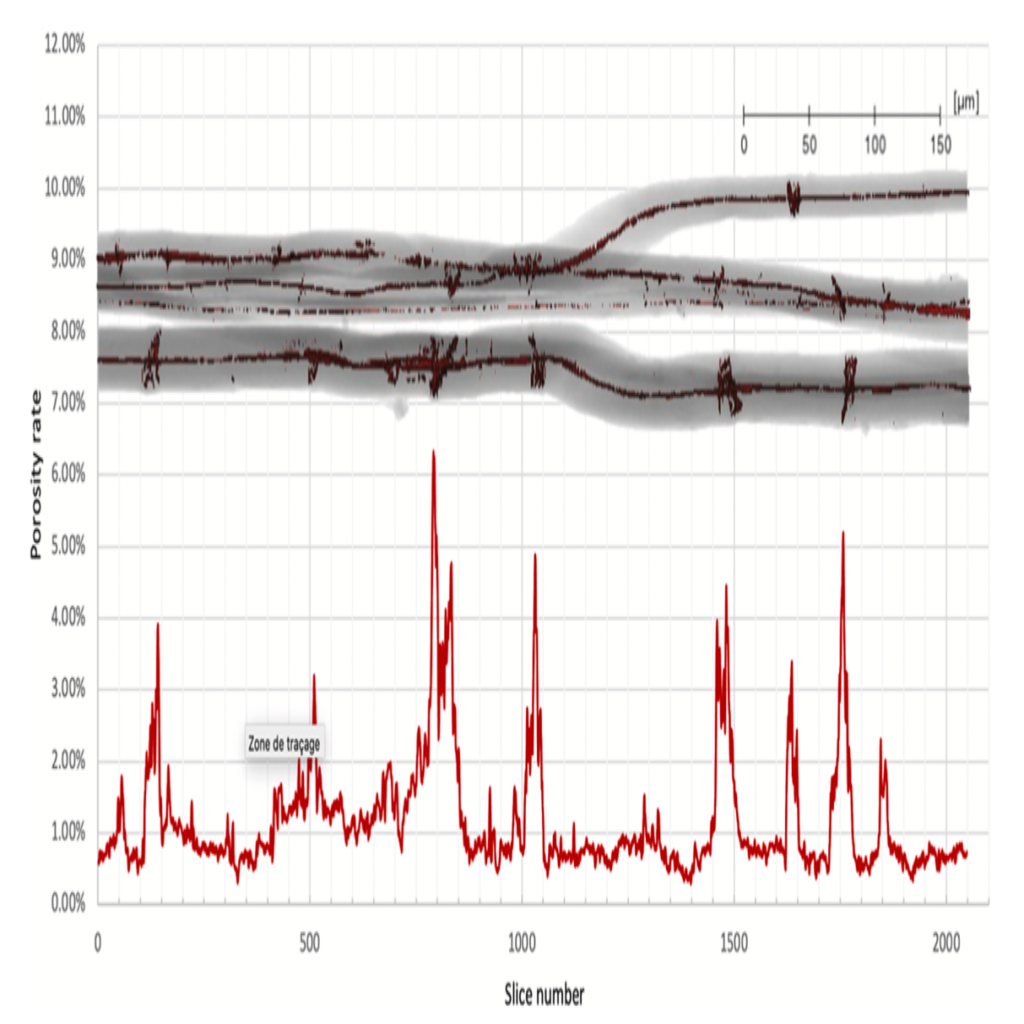
Figure 7. 3D reconstruction of a flax fibre bundle after tomography. Determination of porosities in defect areas
Overall assessment, continuation of the project and collaboration with SOLEIL
Throughout FLOWER, the collaboration with SOLEIL has been constant and has allowed us to develop very innovative and scientifically advanced technologies and knowledge, through the work of Emmanuelle Richely and Alessia Melelli, we have also been able to promote these results in high-impact scientific journals that are internationally recognised in our community.
The data collected was then integrated into the scale of the reinforcements developed within the framework of the FLOWER project, with the aim of gaining a better understanding of those developed and produced by Teillage Vandecandelaère. The models developed are used to predict the behaviour of future materials, prior to experimental tests.
The ultimate aim is to facilitate and increase, in the medium term, the industrial development of plant fibre composites in the cross-Channel area in particular.
Thus, the collaboration with the Synchrotron SOLEIL has been fundamental to the success of the FLOWER project.
- On the one hand, the doctoral students were able to deepen their work, broaden their scientific knowledge, and highlight the link between the structure and composition of flax fibres and the final properties of composites.
- On the other hand, the knowledge of these properties allowed the improvement of the prototypes of the project.
This collaboration is continuing, in particular through the ANUBIS project, financed by the ANR and led by the UBS. It is specifically dedicated to the study of ancient flax and extends until 2025. Note that INRAE and the SOLEIL Synchrotron are partners in this project. Our collaboration with Soleil continues, in particular with Alessia Melelli who ensures the link thanks to her post-doc position at Soleil until April 2024.
[1] The center Synchrotron SOLEIL is made of 29 beamlines, or experimental facilities. They consist of one or several successive cabins where the beam is propagated from the storage ring, selected, focused, and directed toward the samples being studied. Each beamline is specialized and equipped for one or a few techniques of analysis. At SOLEIL, the synchrotron radiation ranges from far infrared to hard X-rays. In this article, we will focus on the beamlines DISCO, SWING and ANATOMIX.